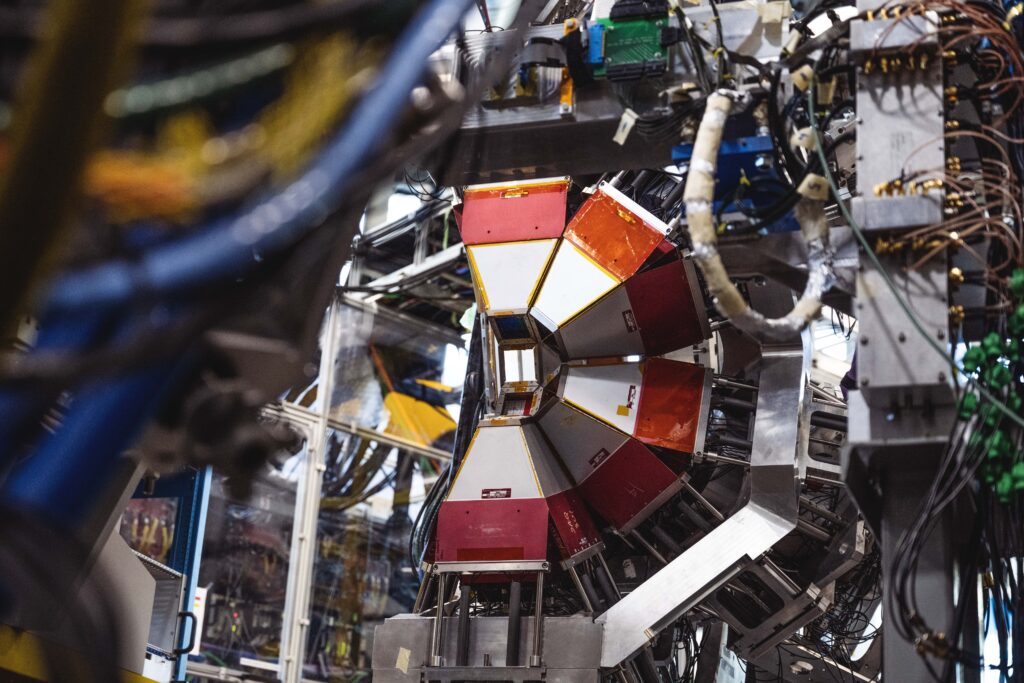
The elements in our Universe are produced in unthinkably extreme conditions. On the Periodic Table, all elements up to iron are forged in the fiery cores of stars; everything beyond must be produced in an even more powerful event – like the collision of two neutron stars.
The catastrophic nature of the elements’ origins makes these reactions very difficult to study on Earth. The exact mechanisms that dictate element production pathways – the specific interactions that the atoms experience as they mix and coalesce and unite within star cores or mergers – are not yet fully understood.
Now, a multidisciplinary study led by Ernest Rutherford Fellow Dr. Matthew Williams at the University of Surrey, alongside collaborators at the University of York, Materials Science Institute of Seville (CSIC-Univ. Seville, and TRIUMF has pioneered a new way to measure nuclear reactions using new nanomaterials.
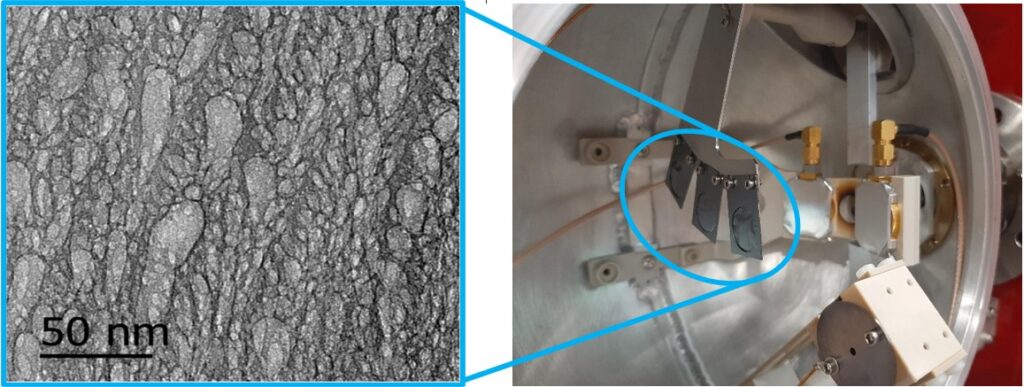
The study, published in Physical Review Letters, leveraged the combined capabilities of TRIUMF’s EMMA and TIGRESS facilities to measure a particular set of nuclear reactions key to understanding whether the element production patterns observed in the oldest known stars match those expected to be produced by merging neutron stars – like the event detected in 2017 by the LIGO gravitational wave observatory.
The experiment involved firing a beam of radioactive strontium-94, produced at the TRIUMF facility in Canada, onto a novel nanomaterial target to induce a key reaction that is predicted to occur when two neutron stars merge. These experimentally produced strontium-helium fusion reactions are thought to occur inside fast-moving winds of material ejected from merging neutron stars or supernovae (exploding massive stars), providing researchers with deep insight into the reaction conditions. The extremely hot winds are thought to be one of the few places in the Universe where elements heavier than iron can be forged, making them an ideal testbed for characterizing the element production patterns that contribute to the heavier elements on the Periodic Table.
The critical tool for this reaction is the new target technology: a thin-film of silicon permeated by billions of nano-bubbles, each approximately 10,000 times smaller than the width of a human hair and filled with helium. Created by a team at the University of Seville, these targets pack more helium into a smaller space than any traditional method, boosting the chance that an inbound strontium-94 nucleus fuses with the helium.
Results from this experiment indicate the strontium-helium fusions reactions do not proceed as quickly as predicted by theory, which has significant downstream impacts on which elements can be formed in subsequent reactions. More work will be carried out to determine whether computational models of neutron star mergers and supernovae, now with updated nuclear physics information, can reproduce elemental signatures seen in very old stars.
“Understanding the origin of elements heavier than iron is one of the big puzzles in nuclear physics and astrophysics,” explained Williams. “We simply don’t have all the information needed to know precisely where and how these elements were made, in part because the relevant nuclear reactions are extremely difficult to measure. In this study, we utilized advances in materials science to get around problems in making compact helium targets. This allowed us to measure for the first time reactions that fuse helium with radioactive nuclei produced in explosions caused by neutron star mergers and supernovae, which are candidates for where heavy elements can be made. These results will open up more opportunities to measure similar types of reactions in the future, hopefully leading us towards clearer picture of how heavy elements are made and role of neutron star mergers in this long-standing puzzle.”
Congratulations to the experimental teams!