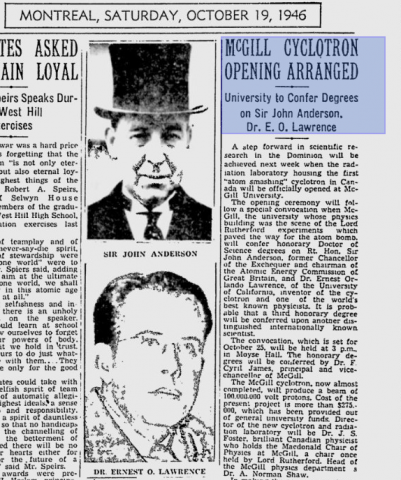
As the dark, cold days of January descend upon us, many people wish they could go into hibernation – or at least escape to the south – until spring rolls around. Even TRIUMF's cyclotron takes time off during this period, shutting down between December and March for its annual maintenance regimen. Among other regulatory tasks, yearly repair to the extraction and diagnostic probes is needed to keep the equipment in top shape as it celebrates its 40th year in operation. As the cyclotron lid is lifted and a team of engineers and technicians begin their work, we take a look back at how far cyclotrons have come since their invention less than 100 years ago.
In 1919, a milestone was reached in nuclear physics as Ernest Rutherford bombarded the nucleus of nitrogen with alpha particles from a radioactive source, causing it to transform into a nucleus of oxygen and emit a proton. This was the first documented instance of artificial transmutation between elements, and it opened many new avenues for research. In order to undertake a full range of experiments, however, a larger supply of more energetic particles was necessary. Researchers across the world responded by inventing and building particle accelerators.
One young physicist from Berkeley, Ernest Lawrence, conceived of a novel method for particle acceleration, which would overcome the challenge of maintaining high enough voltage to induce nuclear reactions – expected to be more than 1 MV. He proposed the use of a lower voltage more than once, using electrodes whose voltage alternated at radio frequencies. Magnetic fields would be used to bend the ions' path so they repeatedly passed over the same electrodes, gaining energy each time. This design was successfully implemented in 1931, as the world's first cyclotron, only 4 inches in diameter, accelerated hydrogen ions to 80 keV energy (as if they had made a single passage across 80 kV) using only 2-kV electrodes.
Cyclotron technology and capabilities advanced rapidly, offering huge potential for the production, detection, and exploration of new radioactive isotopes. The device spread quickly to other universities, and in 1938, McGill University laid plans to build their own cyclotron. McGill's expertise in the field of physics was already well established, having been the site of Rutherford's Nobel Prize-winning research on radioactivity between 1898 and 1907, as well as other significant discoveries.
![]() | Their plans were put on hold with the onset of the Second World War, as key scientists around the world were recruited for war efforts and funding for the project was put on hold. With the war's conclusion, plans resumed, but this time with a major change motivated by wartime advances in radiofrequency technology. Early cyclotrons, operating at fixed frequency, were limited in energy to 10-20 MeV due to a relativistic effect, the increase ineffective particle mass (m) in proportion to total energy (E) mandated by Einstein's famous equation E= mc2. By varying the radiofrequency during acceleration to compensate this effect, synchrocyclotrons were capable of producing beams of much higher energies. On October 25, 1946, a celebration
was held to inaugurate construction of Canada's first "atom-smashing
cyclotron". During the opening ceremony, an honorary degree was
conferred upon the cyclotron's inventor, Lawrence. Hailed in the
newspapers as "a step forward in scientific research in the Dominion,"
McGill's synchrocyclotron cost $275,000 to build. By 1949, it was fully
operational, accelerating protons to an energy of 100 MeV. The synchrocyclotron was hampered by the fact that it could only produce beams in individual batches rather than continuously, resulting in low (time-averaged) beam intensity. By the mid-1950s, cyclotron designers had created an improved cyclotron design, which used azimuthal variation in the magnetic field instead of frequency-modulation to enable particles to be accelerated to higher energies. Further refinement of this design led to the spiral-sector cyclotron, which used spiral-shaped magnets to improve beam focusing and reach even higher energies. This design was pioneered by Reginald Richardson at Berkeley and UCLA, and implemented at various institutions, including the University of Manitoba in 1964. |
In 1965, Richardson's design for a very-high-energy cyclotron was adopted by a group of nuclear physicists from British Columbia who planned to build a high-intensity source of pions and muons. Serving as second director of TRIUMF from 1971‑5, Richardson (himself a native of Canada) completed the construction of the world's largest cyclotron, which would become the cornerstone of TRIUMF's operations. Capable of accelerating hydrogen ions to 520 MeV, the TRIUMF cyclotron produced its first proton beam in 1974, greatly extending the capacity for particle and nuclear physics research in Canada. In 1978, TRIUMF entered into its first commercial partnership with AECL Commercial Products (now known as Nordion), working together on the development of cyclotrons for medical radioisotopes. In addition to this fruitful collaboration, TRIUMF has also partnered with companies such as Advanced Cyclotron Systems Inc. (ACSI of Richmond, BC) and TRIUMF-supported spin-off companies. Nordion now has three small cyclotrons in operation at TRIUMF, and many more have been installed at hospitals and universities across Canada – a high proportion of them being ACSI models. The hospital cyclotrons are used mainly for the production of the medical radioisotopes used in PET/CT scans, but could eventually provide local sources for the most commonly used isotope, Tc-99m, for SPECT scans. | Beam opening, TRIUMF, 1974. |
Canada's place at the forefront of cyclotron technology, which was established in 1946 at McGill, now continues at TRIUMF. Bringing Canada's cyclotron legacy full circle, McGill University joined the TRIUMF consortium as an associate member in 2013. Now the pace of accelerator development continues with the construction of a new superconducting electron accelerator in the ARIEL facility, which is slated to open later this year. This will expand Canada's capabilities to produce and study isotopes for physics and medicine, ensuring our status as a leader in particle and nuclear physics as we work together to discover what the future has in store.
-Lindsay Kroes, Communications Assistant