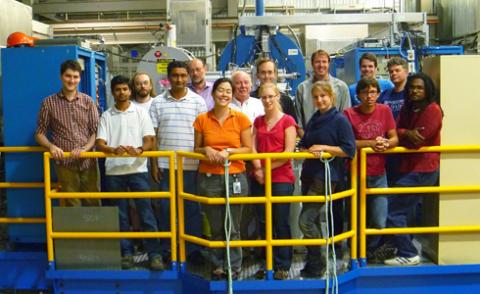
Ingenuity is the cornerstone of physics – who would think that a solution to a present problem might lie, not in a novel discovery, but in questioning the accepted ways of the past? Dieter Frekers and the TITAN team used this idea as a basis in attempting to explain the discrepancy that arose out of the SAGE and GALLEX solar neutrino experiments, in which the number of neutrinos detected was 10-15% less than expected. The experiment proposed by Freker’s was conducted at TRIUMF’s TITAN facility to test the accepted Q-value for the germanium (71Ge) electron capture process – a value that factors into determining the number of expected neutrino events.
Photo (right): The TITAN Group standing by the experiment in TRIUMF's ISAC-I Facility.
Background
The need for precisely measuring the Q-value stems from SAGE and GALLEX solar neutrino experiments. In these experiments, as a (solar) neutrino enters the gallium-71 (71Ga) filled detector, it may collide with one of the 71Ga atoms causing it to convert to a 71Ge atom. The presence of the 71Ge is detected through the distinct X-ray fingerprint it leaves as it decays, and each 71Ge atom present corresponds to a single neutrino presence. Both experiments showed that the number of neutrinos detected was roughly half of that expected, leading to the discovery of neutrino oscillations.
To ensure that the detectors were functioning properly, SAGE and GALLEX needed to be calibrated. This involved putting an intense terrestrial neutrino source, in this case chromium-51, at the centre of the detector and comparing the measured events to the expected ones – a ratio that should be one, since both the strength of the source and the response of the detector were precisely known. Regardless, a 10 – 15% discrepancy persisted.
Reviewing the Q-value
It was then that Dieter Frekers and co. decided to take a closer look at the neutrino cross section – a key part of the equation devised by the “Grand Seigneur of solar neutrino physics”, the late John Bahcall.
“The experiment was conceived while we were in Japan last May, discussing our results that we had just measured at Osaka – we couldn’t make sense of them …. after some intense discussion, we decided that we wanted to look more carefully at the Q-value” comments Frekers. The Q-value is the mass difference between two nuclei (71Ge and 71Ga), and it enters quadratically into the calculation of the cross section (as illustrated below).
The diagram illustrates how the Q-value contributes quadratically to the cross section
A literature review revealed something astonishing – the Q-value presently used had never been measured directly. It was a side-effect of previous experiments that had looked at other phenomena, such as the Bremsstrahlung component of electron capture or the infamous 17 keV neutrino, which caused some hype in the late '80s. If the Q-value were to change by about 7%, that would be enough to eliminate the discrepancy! It was clear to Frekers and his team that an experiment designed specifically to measure the Q-value was warranted. A direct mass measurement in a Penning trap could settle this debate, since this method delivers accurate and precise results.
Experiment
The experiment took place at TRIUMF’s TITAN facility at ISAC over the course of six days, August 12-18. TITAN is an ion trap system for high precision mass measurements with unique features: It is the fastest system of this type in the world and the only one at a rare isotope facility, where the ions can be brought to high charge states before the actual measurement in the Penning trap.
This charge state boost happens in an electron beam ion trap that was built in collaboration with the Max-Planck-Institute in Heidelberg and it was crucial for this Q-value experiment in two ways. Firstly, the precision of the measurement scales linearly with the charge state of the ion. Secondly, and this was a first, it allowed for the separation of nuclei with the same nominal mass (so call isobars) based on their different atomic structure. More strictly speaking, in the neon-like charge state (i.e. a nucleus with ten electrons left) Ga is a 21-times and Ge a 22-times charged ion and hence can easily be distinguished. The electron beam ion trap was tuned such that no Ge23+ or Ga22+ was produced, which means, that a pure germanium-beam of mass 71 and charge state 22 could be produced. An extra trick came in handy from the laser ion source group at ISAC, where a pure gallium-beam was made available, since the ionization of Ge happens only when using a very specific laser ionization scheme. While Ga ions are always produced through surface ionization, the admixture of Ge could be switched on and off easily with the lasers.
The bulk of the measurements were performed by inserting a small number of 71Ga21+ or 71Ge22+ into the Penning trap, where a radiofrequency (rf) was then applied. Using a certain type of rf-excitation the trapped ions will gain energy only if the applied frequency is close to the so called cyclotron frequency, which is proportional to the charge-to-mass ratio of the ion. The ions are extracted from the Penning trap after this excitation and an energy gain can be detected as a decreased time of flight. By scanning through the frequencies one finds the cyclotron frequency extremely accurately at the minimal time of flight.
The laser ionization scheme for Ge worked so well, that Ge ions form ISAC could be delivered to TITAN in similar amounts as Ga ions. This surpassed the expected yields by a factor of about 100. As a consequence it was possible to perform an independent cross check measurement on the mixed beam of Ga and Ge in charge state 21+. A time of flight frequency spectrum obtained in this configuration is shown below. As the ratio of the two frequencies is inversely proportional to the mass ratio, the Q-value could then be determined.
Results
The results were the really interesting part, because contrary to what Frekers hoped for, they showed that the Q-value measured at TITAN was surprisingly close to the one used by Bahcall (232.5 keV). So the question arises – if it’s not the detector (SAGE or GALLEX), nor the calibration or the neutrino cross section, what is it that accounts for the discrepancy?
The difference in frequencies yields the Q-value
This experiment has spawned many new questions – the primary one being about the existence of ‘sterile neutrinos’, a flavor which makes neutrinos blind to any known interaction. Their presence may help explain the discrepancy as it would decrease the cross section; however, much research is yet to be done before we will know all there is to know about neutrinos.
As pointed out by Dieter Frekers (Uni Muenster) himself, who led the international collaboration and has been studying neutrinos for most of his career – “neutrinos are always up for surprises, and fascinatingly enough almost none of the theoretical predictions over the last 30 years concerning neutrinos were correct, they were simply all proven wrong by an experiment.”
Only time will tell what the next revelation will be!
Written by Lena Sitnikova, Outreach Assistant